Do electric cars use more water than petrol cars?
With current electricity mixes, yes. In a low-carbon world, no.
I would have guessed that electric cars used more water than petrol ones because mining critical minerals is a water-intensive process. I assumed this increased water use was an inevitable trade-off.
But the data is more nuanced.
The majority of water used over a car's lifetime comes from the fuel – whether it's electricity or oil – not the car's production. An electric car running on fossil-fuel-powered electricity indeed uses more water than a petrol car. But when powered by low-carbon sources, especially solar and wind, it uses much less.
Transitioning to electric cars and cleaning up our grids will reduce water use overall.
That said, mining for critical minerals can exacerbate water stress in specific regions. Both points can coexist: global water use for energy and transport could decrease, while localised water supplies may face increased strain.
Let’s look at the data.
Solar and wind use much less water than fossil fuels and nuclear
Thermal power plants – like coal and nuclear – use large amounts of water for cooling. That means use much more water than sources such as solar PV and wind.
In the chart below you can see the average water consumption of electricity sources per megawatt-hour. This is the median value from a meta-analysis by Yi Jin et al. (2019), which included 32 studies.1 This is based on the life-cycle of the plants, so includes water use in supply chains, not just the plant itself.
Biomass uses the most, followed by hydropower, then nuclear and coal. Natural gas still uses more than solar PV and wind, but is more water-efficient than coal.
This mirrors the rankings from other studies. The UNECE, for example, carried out out a large analysis of the life-cycle impacts of electricity generation in Europe. It produced similar results for water consumption: nuclear uses slightly more than coal, followed by gas, then solar PV and wind.
There can be large differences in water consumption for a single electricity source across different regions, and in the case of thermal power plants, the type of cooling system that’s used.
I’ve included the chart below – from the Yin et al. (2019) paper – which includes the full range estimates for each electricity source. Note that this is on a logarithmic scale. You can see that some span orders of magnitudes, in particular for sources such as hydropower and biomass. Sources such as nuclear and coal have very strong overlap.
The main conclusion, though, is that solar PV and wind tend to use much less water than coal, gas (although to a lesser extent) and nuclear.
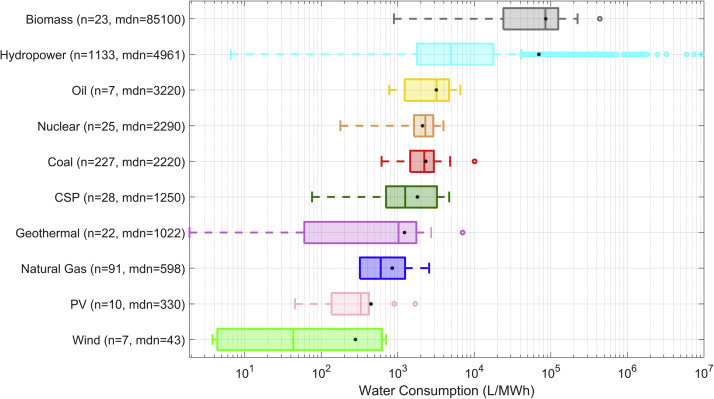
Most electric cars today use more water than petrol ones, but this will change with decarbonisation
Back to the main question of electric cars. You’ll soon see why the comparisons of electricity sources was an important detour.
Most electric cars on the road today use more water than their gasoline equivalents. But this isn’t because of the water use for critical minerals or building the car. It’s mostly about the electricity to run it.
A study by Wang et al. (2020) looked at the life-cycle water use of two cars in China and the United States. It compared the Ford Focus car with a combustion engine to the Ford Focus electric. Obviously the values will vary if you’re comparing different models of car, and cars of different sizes.
It found that the gasoline car used around 137 cubic metres (m³) of water over its lifetime. The electric car used almost twice as much: 262 m³. The electric car was more water-efficient in the US, using 170 m³, but this was still more than the gasoline equivalent.2
The fuel accounted for 72% of the water use for the gasoline car. The electricity generation made up 82% for the electric car. It’s the extraction of (and refinement of) the gasoline and power generation that matters most. An earlier study by Kim et al. (2016) found very similar results.3
This explains why an electric car in the US would use less water than in China. Remember the electricity source chart we looked at earlier? China’s electricity grid uses much more coal and hydropower than the US’s grid does, and both of those sources use a lot of water. Water use for electricity in the US has been falling in recent decades as it moves away from coal to natural gas and renewables.
Of course, we’re trying to decarbonise our electricity grids so the water footprint of electric cars is going to change over time. How would their water use compare if the electricity was generated from solar or wind, for example?
You can see the comparison in the chart below. Again, you see that an EV running on China’s current electricity grid uses more water than a gasoline car. But if it was run on solar or wind power, it would need much less. That’s because both power sources use little water.
Moving to electric cars only makes sense from a water perspective if we’re decarbonising the grid at the same time. Even then, this is only really true if most electricity is coming from solar or wind (or geothermal). In grids dominated by nuclear power, water use could actually go up, not down.
Water stress for critical minerals is a serious but highly localised problem
So, electric cars could use less water than gasoline ones, if powered by renewables. And generating the power often uses more water than the mineral extraction and production of the vehicle itself.
How does this tally with reports of intense water stress for mining critical minerals?
Well, both points can be true at the same time. That’s because scale matters. Water use can go down at the aggregate level, while also increasing water stress in particular localised areas.
This is why you get the International Energy Agency publishing in-depth reports on the risks of minerals and water stress, and reports titled “Clean energy can help to ease the water crisis” at the same time.
In the chart below, you can see how water-stressed current production of different minerals is. Lithium is perhaps the most relevant mineral for electric cars since the industry is dominated by lithium-ion batteries.
More than half of the world’s lithium is produced in areas of “high” or “extremely high” water stress.
It’s important to clarify what this means. Water stress is calculated from two metrics: how much water is available on the surface and underground and how much humans are extracting out of the ground each year. To make sure we keep ample supplies, we want to extract only a fraction of what is available underneath us. Regions with “extremely high” stress are extracting more than 80% of what is available; those with “high” stress are extracting more than 40%. So, having “high” water stress doesn’t necessarily mean you’re on the brink of running out. But it does mean that you’re extracting a lot compared to how much is available and replenished.
The water demands of lithium depend on how it’s produced. Most lithium extracted in Australia comes from ‘hard rock mining’; basically digging minerals out of solid ores.
But lithium production in the “lithium triangle” – Chile, Argentina and Bolivia – comes from brine. Salty liquid from underground is pumped to the surface and left in open pools to evaporate. Lithium and other minerals are then left behind when the water has disappeared. This method uses a lot of water and is highly localised in areas that are already under high levels of water stress.
This is a significant concern as demand for lithium increases, especially if it competes with demand for agriculture and basic water supplies for households.
But again, even in water-stressed Chile, it is a very localised problem. There, just 4% of water consumption is used for mining. 72% is used for farming.
The problem with mining is that it’s highly concentrated in specific locales. Mining uses a much larger share of water in northern Chile, and in particular regions such as Antofagasta, it uses more than half of overall water consumption.4
How can we reduce water stress for minerals such as lithium?
We shouldn’t forget about the real water stress issues involved in mining, even if total water consumption goes down with clean energy and transport.
What can be done to relieve water stress in industries such as lithium?
There is a very obvious solution – which I probably sound like a broken record on – to reduce demand for lithium. Recycling will be crucial. Investing in public transport, encouraging walking and cycling, and dampening demand for private car ownership. Maybe even shifting towards battery technologies that don’t use lithium, such as sodium-ion.
All of these things will help to relieve stress on supplies but won’t eliminate it completely. The world is going to need more lithium in the years ahead, so we’d better also find a more sustainable way of mining it.
If the problem of water stress is about intense localisation, then diversifying supplies would go a long way. Less lithium from South America and more from Australia (which doesn’t rely on brine) and elsewhere.
But we can also improve water management in places like Chile. The government there has committed to ensuring that mining uses less than 10% of water in 2025 and less than 5% in 2040, despite the rapidly growing demand for these minerals.
Desalinisation is one option (which I might cover in a future post). Investments in water repurposing and reuse can help. Some experimental technologies are developing, too. “Direct lithium extraction” captures lithium directly from the brine without having to evaporate all of the water.
These are essential investments for mining companies as well as other companies within the lithium and battery supply chains. If we take this problem seriously and develop good and cost-effective solutions, then a move to clean energy can reduce water demand at a global level while also alleviating water stress in those communities extracting the minerals to power it.
Jin, Y., Behrens, P., Tukker, A., & Scherer, L. (2019). Water use of electricity technologies: A global meta-analysis. Renewable and Sustainable Energy Reviews.
Note that the study assumed – for simplicity – that the driving distances in China and the US were the same i.e. the average driver racked up the same mileage over the lifetime of the car.
That's not the case: the average mileage in the US is likely to be much higher.
Kim, H. C., Wallington, T. J., Mueller, S. A., Bras, B., Guldberg, T., & Tejada, F. (2016). Life cycle water use of ford focus gasoline and ford focus electric vehicles. Journal of Industrial Ecology.
Garcia-Zavala et al. (2019). An approach for prioritising environmental, social and governance (ESG) water-related risks for the mining industry: The case of Chile. The Extractive Industries and Society.
Thank you. Another insightful post. One question. Is all water consumption equal in impact? For example, does using water for cooling thermal electricity plants and hydropower - where the water is diverted and reused - have less impact than water used for biomass production or materials processing? Would allowing for any difference in impact affect the outcome of the analysis? Thanks.
Since agriculture is by far the biggest source of water consumption, doesn't it make more sense to promote water efficiency there instead. Perhaps promoting drip irrigation and water pricing.