We have enough minerals for the energy transition, but medium-term supply is a challenge [Part 1]
We won't run out of minerals to build a low-carbon energy system.
In a recent article, I looked at the mineral requirements for moving to low-carbon electricity systems.1 In short: we have enough to decarbonise our electricity systems.
But that work didn’t include electric vehicles, grids, and other smaller parts of the transition. This could make a big difference to the results: EVs, for example, are large users of minerals such as lithium, cobalt, and copper.
Here I’ll look at total supply and demand for key minerals.
But first I wanted to give a word of caution: no one knows exactly how much of each mineral we’ll need. We don’t know how chemistry batteries will change by 2050; how much solar or we’ll have; or our total energy demand.
There are other reasons why I’m hesitant to analyze ‘will we have enough minerals?’ based on supply and demand projections. These metrics are never static. Markets are very good at giving incentives so that we don’t run out.
First, when we go looking for something, we tend to find more of it. Crazy, right? Decades of false predictions about ‘peak oil’ are evidence of this. Lithium reserves and resources have increased over time, despite us using more of it. There has been a stream of headlines in recent months about ‘new discoveries’ of mineral deposits.
Second, manufacturers are often good at substituting materials when supplies seem tight. Prices of one mineral go up, so they substitute for another. The chart below – from the Energy Transitions Commission (ETC) – gives two examples of this.
On the left, we see that projections for cobalt have fallen a lot as some electric car manufacturers have moved to cobalt-free chemistries. On the right, we can see how high copper prices drive a shift towards aluminum.
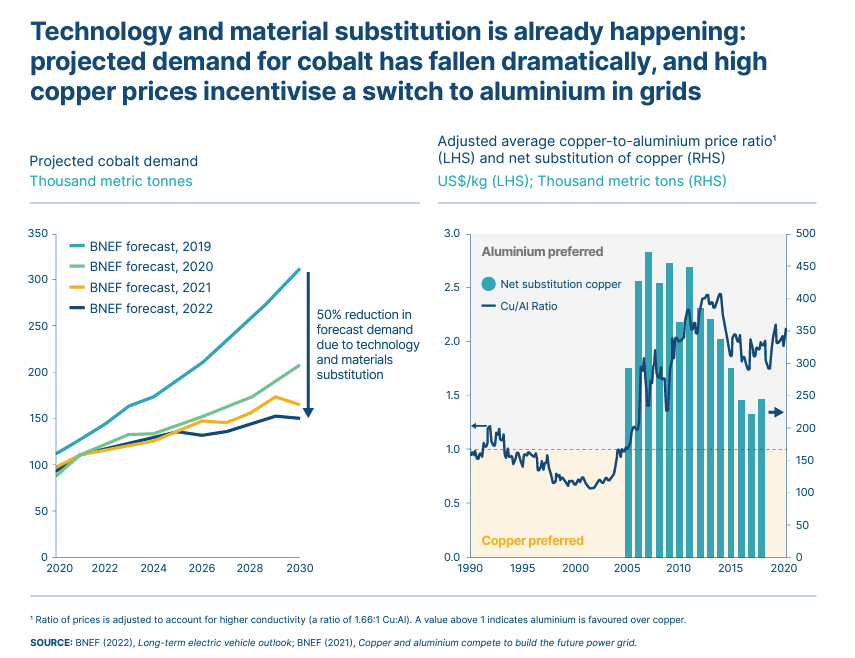
That’s why a simple supply-demand projection several decades into the future is never going to give us an exact answer.
But I know that most people won’t be satisfied with: “we don’t know, but I’m sure markets will sort it out”.
We can still put sensible estimates on these numbers, and they’ll give us some useful information. I wouldn’t panic over a supply-demand mismatch of 20% (because we expect that we’ll work it out through exploration and material substitution). But if we’re projecting mineral demand that’s 5 or 10 times more than our resources, then it’d be useful to know that now…
That’s what we’ll look at here.
Will we run out of key minerals by 2050?
I’ll first focus on a large report – Material and Resource Requirements for the Energy Transition – published by the Energy Transition Commission (ETC). It’s a great report that’s recommended reading if you’re interested in this area.
We would want to look at projections from various organisations, rather than relying on one. So, I’ll then look at how other projections compare.
The study looks at mineral requirements from 2022 through to 2050.
It’s based on an ambitious scenario that reaches net-zero by 2050.2 The world’s electricity is decarbonised, there is a near-total decarbonisation of passenger transport, heavy industry is supplied by green hydrogen, and it assumes 7-10 GtCO2 of carbon capture and storage for the remaining emissions.
Let’s look at the summary provided by ETC, then we’ll look at six key minerals individually.
In the chart below, ETC splits minerals and bulk materials into those where reserves and resources are more than sufficient to meet demand (in blue, on the left) and those where the gap is much closer (in green, on the right).
The dark blue bar for each mineral shows the cumulative demand to 2050. This is not just demand for low-carbon energy, but for all non-energy industries too.
The light blue bar is the estimated reserves. Reserves are deposits that we can extract economically with current technologies.
Resources are known deposits but include those that are economically viable to extract. They are shown as green bars. Reserves and resources can both increase over time as we discover more and as deposits become economically viable.
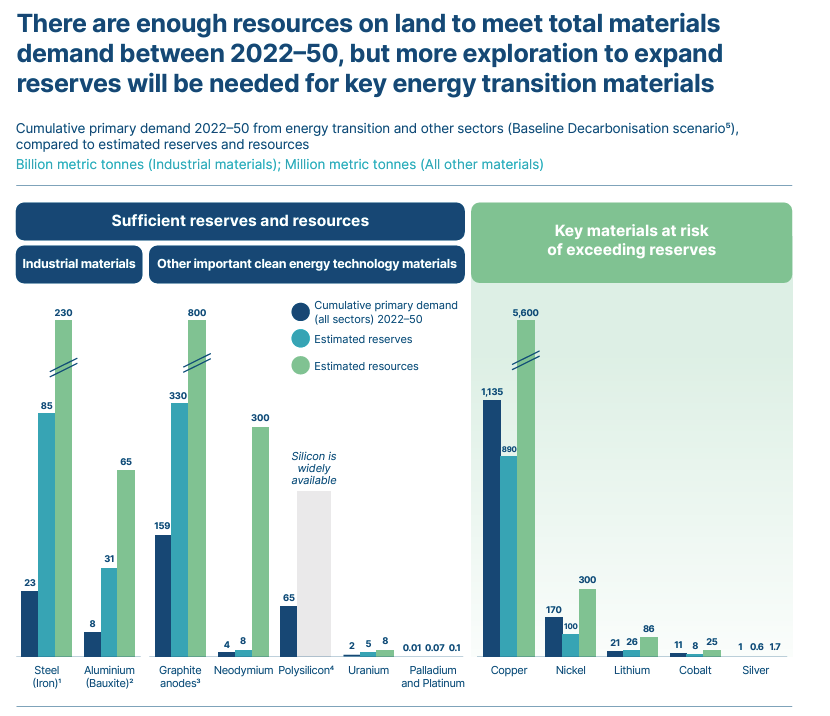
The materials on the left-hand side of the chart are not a concern: demand is well below our current reserves.
On the right, you can the minerals where demand is higher than or close to current reserves, but lower than our known resources. This means that the world does have enough copper, nickel, lithium, cobalt, and silver, but we will need to make more of these economically viable or find new deposits that are easily accessible.
Mineral-by-mineral
Lithium
Lithium is a dominant mineral in electric vehicles, but is also used in stationary batteries.
The ETC estimates that from now until 2050, a net-zero scenario would need around 19.5 million tonnes of lithium. We’ll also use around 2 million tonnes for non-energy purposes, so total demand will be 21.5 million tonnes.
With smaller batteries, a switch to other battery chemistries, and improved recycling, this could be reduced to 12 million tonnes.
The world has enough lithium to meet this demand in the long-term.
We have around 26 million tonnes of lithium in reserves. Remember, reserves are deposits that we can extract economically with current technologies.
There is much more in resources – 86 million tonnes.
Reserves and resources can both increase over time as we discover more, and as deposits become economically viable. In a previous post, I showed that both metrics for lithium have increased over time.
Cobalt
Cobalt has been used in a range of products. It’s used in lithium-ion batteries, so has been a key component in consumer electronics for decades. Electric cars are now the largest user of cobalt.
The ETC estimates that cumulative demand by 2050 will be just over 11 million tonnes.
In a reduced demand scenario, this falls to 7 million tonnes. This would involve a move away from cobalt-rich chemistries: either to low-cobalt NMCs, lithium-iron phosphate, or sodium-ion batteries.
Some manufacturers are already making this move: Tesla has been shifting towards lithium-iron phosphate, and sodium-ion batteries are growing among Chinese producers.
Current reserves (at 8 million tonnes) are insufficient to meet our 2050 demand in a baseline scenario. So, we would need to reduce demand or find new economically-viable deposits. Resources are more than sufficient to meet demand if they can become viable.
Child labour and poor working conditions is a major problem in cobalt supply chains in the Democratic Republic of Congo. I covered this issue in detail in an earlier post. These issues could accelerate the battery shift away from cobalt.
Copper
Copper is used in a range of low-carbon technologies: solar, wind, electricity grids, and vehicles.
In most processes, it’s hard to substitute, although aluminum could be substituted in some grid applications.
As you can see from the chart, current reserves will need to expand to meet demand in 2050. The world has large quantities of copper in resources, but these can be hard to extract or are in very low concentrations in ores.
Graphite
Graphite is used in batteries, so electric cars are the primary driver of demand.
As we can see in the chart, demand to 2050 is well below global reserves of natural graphite. Graphite can also be produced synthetically, so it’s unlikely the world would run out of supply in the long-term.
Neodymium
Neodymium is an important component in high-strength magnets and is used in electric car motors, and wind turbines.
Demand could be reduced through recycling at the end-of-life, or possible shifts in motors away from technologies that need rare earths.
As we can see in the chart, the world has sufficient reserves to meet demand through to 2050.
Nickel
Nickel is widely used in non-energy sectors.
In low-carbon energy, it’s used in electric cars, hydrogen electrolysers, and wind power.
A shift away from cobalt-rich batteries has already led to an increase in demand for high-nickel chemistries. Demand for nickel would be reduced if battery chemistries were to shift again towards lithium-iron phosphate or sodium-ion.
As we can see in the chart, reserves would have to expand by 2050. Global resources are sufficient to meet this demand.
ETC estimates of mineral demand are high compared to other analyses
We might be concerned about basing our summary on this ETC report alone. Maybe it assumes an artificially low demand for minerals.
We can cross-check these assumptions with other analyses. When we do, we find that ETC usually has the highest mineral demand. That should give us even more confidence that we’ll have enough.
The Payne Institute, led by Morgan Bazilian at the Colorado School of Mines produced a State of Critical Minerals report earlier this year. It’s worth a read if you’re interested in this topic; it covers a range of issues including geopolitical, environmental, and social implications of mining.
In it, they looked at the range of estimates from organisations on future mineral demand. I’ve included the chart for copper below.
As you can see, the ETC estimate – shown as the dark blue line – trends high compared to other estimates. Its cumulative demand figure – which would be the area under that curve – will be much higher than most others. The same is true for nickel (see the footnote for the chart).3
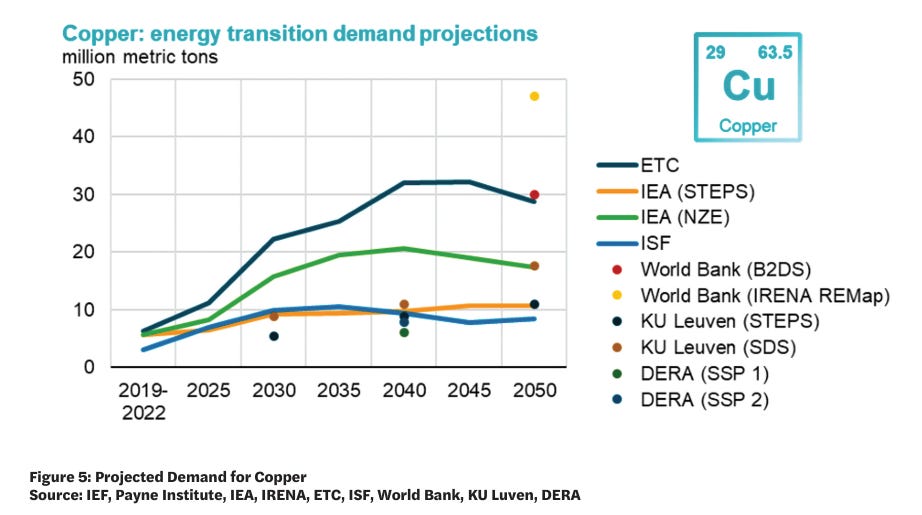
But this is not the case for lithium (see below). The IEA’s Net-Zero scenario trends around 20% to 25% higher through to 2050.
We might then want to account for this in our demand assumptions. Earlier we saw that ETC’s high-demand scenario was 21.5 million tonnes by 2050. We might assume that this could be up to 25 million tonnes instead. That would still be within current global reserves (although very tightly), and well within global resources.
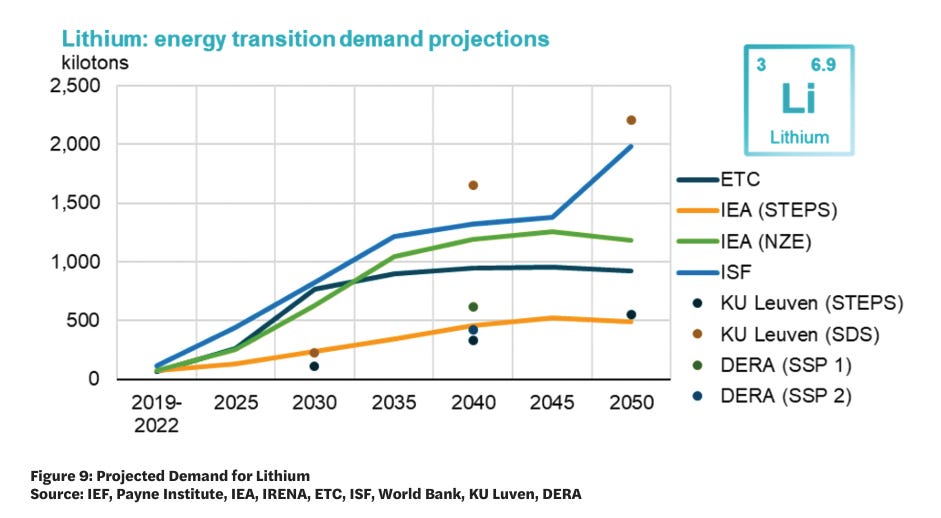
Most demand scenarios will be wrong – but they’re still a useful guide
To reiterate: none of these long-term scenarios will be exactly correct.
They’re used to give a best estimate based on what we know now, and get a broad sense of whether they’re within the bounds of our reserves. They’re used to warn us whether we’re going to be walking into a major bottleneck.
There’s no need to sweat over whether cumulative demand in 2050 is 20 or 21 million tonnes. Doing so overstates our confidence in how things will change over the next 27 years.
But based on the range of demand estimates that I’ve looked at – not just from ETC – I am not concerned about long-term mineral demand. Here are a few examples:
International Energy Agency (IEA): “There are generally no signs of shortages in these areas: despite continued production growth over the past decades, economically viable reserves have been increasing for many energy transition minerals.”
International Renewable Energy Agency (IRENA): “There is no scarcity of reserves for energy transition minerals, but capabilities for mining and refining them are limited. [...] Production has surged for many energy transition minerals, and reserves mined from economically viable sources have grown. Moreover, disruptive innovation - such as efficiency improvements and material substitutions - are already reshaping demand.”
Rocky Mountain Institute: “The world has enough minerals for the entire energy transition, and is rapidly building out the mines to extract them.”
We will have enough to build low-carbon systems.
We should try to reduce demand as much as we can through recycling and material efficiency. For some minerals, we will need to expand our existing reserves by finding economically viable deposits. But the amount of resources that the world has is more than sufficient.
Short-term bottlenecks are a bigger concern. That’s the problem that we’ll look at in Part 2.
This was based on a paper by Seaver Wang and colleagues.
Wang, S., Hausfather, Z., Davis, S., Lloyd, J., Olson, E. B., Liebermann, L., ... & McBride, J. (2023). Future demand for electricity generation materials under different climate mitigation scenarios. Joule, 7(2), 309-332.
ETC has done extensive reports on how to decarbonise each sector, such as Making Mission Possible: Delivering a Net-Zero Economy and Making Clean Electrification Possible: 30 Years to Electrify the Global Economy which includes more detailed analysis of these sectors.
"Copper is used in a range of low-carbon technologies: solar, wind, electricity grids, and vehicles. In most processes, it’s hard to substitute, although aluminium could be substituted in some grid applications." This is where I would LOVE some interviews with engineers etc. Sure aluminium only has 60% of the conductivity of copper. But the following aluminium How would we do it? This is the claim. Just use more! "This means you will need a 25% thicker wire for the same results. However, the aluminium in this equivalent wire will cost and weight about half as much as copper. That’s it. Aluminium in power lines? Of course! Also underground! And in transformers and coils, in motors? Yes!"
He claims over 90% of the roles of copper can be replaced with aluminium, including batteries, EV’s, electric motors, wind turbines, etc. Just use more. https://www.shapesbyhydro.com/en/material-properties/how-we-can-substitute-aluminium-for-copper-in-the-green-transition/
At first, I was trying to understand why electric grid expansion would impact the need for copper since almost all overhead lines are aluminum with steel cores and most of what we need are high voltage overhead transmission lines.
This article from BNEF does a good job of describing the amount of overhead lines versus underground cable that we will need and the amount of copper vs aluminum required.
https://about.bnef.com/blog/a-power-grid-long-enough-to-reach-the-sun-is-key-to-the-climate-fight/
They estimate we will need 427 million metric tons of copper and 650 metric tons of aluminum for the global grid expansion by 2050.
Ideas that could help limit the amount of transmission lines required are storage and repurposing existing fossil fuel plants with advanced nuclear (SMRs) and natural gas with CCS.